At one of the world’s first scientific meetings devoted to a brand new invention called the laser, physicist Peter Franken zoned out.
“I kept hearing, ‘Lasers are fantastic! Eye surgery, communication, eye surgery, communication.’ Eye surgery and communication were all anyone could think about,” the late University of Michigan professor told the American Institute of Physics in an oral history interview. “My mind began wandering.”
Franken started doodling calculations. Soon he was on to something: Lasers could generate electric fields on the order of 100,000 volts per centimeter — not far from the strength of the field that holds atoms together. What would happen if he could crack that field? He kept scribbling. Using what he described as “very simple perturbation theory and dimensional arguments,” Franken came to a revelation.
“I realized, my God, you’ve got a second harmonic!” he said.
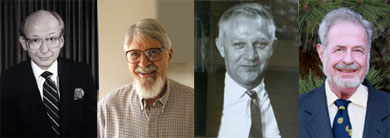
The team of U-M physicists who created non-linear optics. (L-R): Peter Franken stumbled on the idea while bored at a meeting; Gabriel Weinreich figured out which materials to use; Wilbur Peters devised the lab set-up; and Alan Hill, just a sophomore and a tinkerer extraordinaire, ‘made sure the whole thing worked.’
If he sent a laser beam through just the right material, he figured, he could warp the material and coax the beam into overtones, like the harmonic distortions you get from new sound frequencies when you crank up a loudspeaker. He could double the frequency of a light beam. He could turn red light blue. The physicist couldn’t stay in his seat. He left the conference, came home to Ann Arbor, and rented a ruby laser.
That was the spring of 1961. By the end of summer, Franken and a team of Michigan physicists worked together to give rise to a new field of science: nonlinear optics.
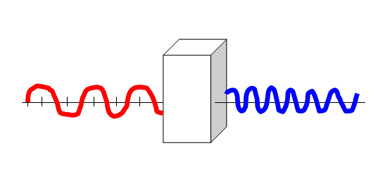
Frequency doubling: A blue light wave has twice the frequency of a red light wave. In other words, it oscillates twice as fast. The shorter the wavelength the smaller the spot the light beam can be focused down to, making possible industrial applications with much greater precision (in addition to many other uses this process makes possible.) (Image courtesy Herbert Winful.)
Gabriel Weinreich, then a new solid-state physics professor, figured out exactly what kind of material the researchers needed to generate the second harmonic. Wilbur Peters, a senior experimentalist, arranged the perfect lab set up. And Alan Hill, a sophomore from Franken’s introductory physics class who had conducted some impressive electromagnetic compression experiments in his parents’ garage, “made things work,” Franken said.
Today, nonlinear optics is a branch of physics that describes how a tightly focused light beam behaves when you push it through certain “nonlinear” materials. If the light is intense enough and the medium has the right structure, you can generate new offshoot beams at wavelengths that didn’t exist in the original. In the past five decades the field has unlocked the electromagnetic spectrum to offer laser frequencies and intensities across the rainbow and beyond, enabling Nobel-winning science and world-changing engineering.
“It’s absolutely amazing what it has led to and what it is leading to,” said Hill, the tinkering sophomore who went on to become chief scientist for high energy laser development at Kirtland Air Force Base in Albuquerque. “It’s helping us understand the nature of nature.”
Thanks to nonlinear optical techniques, scientists have connected the relationship between distance and time in a fundamental way, making it possible to determine the speed of light with zero error. Nonlinear optics can generate a comb of evenly-spaced frequencies that can be used as a kind of optical ruler for measuring time, explained Herbert Winful, a professor in the U-M Department of Electrical Engineering and Computer Science.
“It has also enabled scientists to create light pulses as short as an attosecond — a billionth of a billionth of a second,” he said.
With these pulses that zip across an atom almost as fast as an electron orbits, scientists can examine atomic structure and watch and understand chemical reactions in new ways. Researchers can now use laser light to study and identify materials, answering questions about air quality or the presence of poisonous gases or other hazardous materials, for example.
In long-distance fiber optic communication for Internet, cable television, and telephone applications, a nonlinear optical phenomenon known as stimulated Raman scattering is used to amplify light signals so they can travel thousands of kilometers without degrading.
And the best ground-based telescopes use nonlinear techniques to correct for the blurring effects of Earth’s atmosphere. “Nonlinear optics has had a profound impact on science,” said Elsa Garmire, an engineering professor at Dartmouth who specializes in the field. “It opened up the possibility of relooking at every process having to do with the relationship between light and materials.”
More exciting applications are in the field’s future, researchers say. Quantum entanglement — what Einstein called “spooky action at a distance” — could one day be used in cryptography to create uncrackable codes. New techniques are leading to better ways to identify cancerous cells and perform certain surgeries. Doctors can essentially heat the surface skin so hot and fast that it vaporizes before the underlying tissue gets damaged. Quantum computing, particle accelerators, semiconductors, telecommunications…. Garmire recently wrote a 31-page paper on the field’s applications. Hill, a co-author of the seminal work, never dreamed it would have such broad reach.
“It was purely fundamental physics,” Hill said. “It never dawned on anyone that it would go beyond that point.”
That point. Hill could be speaking literally of the evidence that was actually missing from the printed Physical Review Letters paper. It’s a nifty bit of physics lore. The researchers submitted as proof a photo showing a big splotch where the main red laser beam hit their plate and a tiny dot left by the byproduct second harmonic blue beam. But a meticulous lithographer erased the dot. The journal published the paper with an arrow pointing to an empty space.
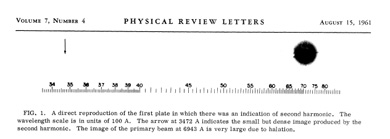
This graphic, from Physical Review Letters, became a part of scientific lore when a lithographer erased the tiny, precise dot showing where a “second harmonic” blue beam was produced, leaving only an arrow pointing to empty space. click image for larger version.
“The whole paper was about the dot,” said Roberto Merlin, U-M’s Peter A. Franken Collegiate Professor of Physics, “and the dot wasn’t there.”
Still, its point was well taken.
The university is hosting a symposium, Nonlinear Optics @ 50, starting at 8 a.m. Wed., Oct. 26 in the Michigan League Ballroom. Speakers include two Nobel Prize winners as well as Alan Hill and Gabriel Weinreich.
Bernard J Leininger MD - 1966 cardio-thoracic
Wonderful story–appreciated by a Non-Physicist
Reply
Sushil Birla - 1997
Very well narrated history. Even though I am far removed from research in physics, it was facinating reading.
Reply